
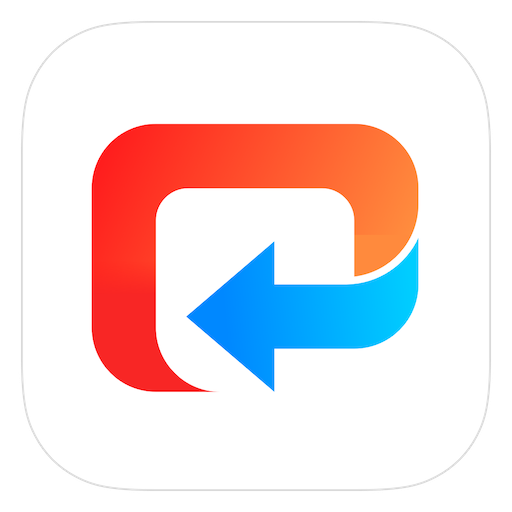
However, despite its unique advantages, many 3D or live-imaging applications in biology remain practically out of reach due to the fast dynamics or the photosensitivity of the biological samples involved. Brillouin microscopy has enabled a wide range of applications in biology, including studies of intracellular biomechanics in living cells 9, of ex vivo 10, 11 and in vivo tissues 12, 13, 14 and their components 15 (for example, collagen and elastin, as well as biomaterials 16), and early disease diagnosis 17. When coupled to a confocal microscope 8, Brillouin microscopy can achieve three-dimensional (3D) diffraction-limited resolution. By analyzing the frequency shift ( v B) and linewidth (Γ B) of the light that is inelastically scattered from gigahertz-frequency longitudinal acoustic vibrations (phonons) in the sample, one can deduce the elastic and viscous properties of the sample, respectively. Recently, Brillouin spectroscopy has emerged as a non-destructive, label- and contact-free technique that can assess the viscoelastic properties of biological samples via photon–phonon scattering interactions 5, 6, 7.

Although molecular components of cells can routinely be visualized with fluorescence microscopy in biology, assessment of the mechanical, that is, elastic and viscous, properties of living cells with similar spatiotemporal resolution is more challenging given the intrinsic limitations of current biophysical techniques 2, 3, 4. The fields of mechanobiology and physical biology have grown quickly in the past decade, revealing that cell and tissue mechanics play an integral role in determining biological function 1.
